The Outlook of Biofuels for Shipping – Opportunities and Challenges
In the recent MEPC 80, the International Maritime Organization (IMO) enhanced the targets for decarbonizing shipping, by mandating a 20% reduction in absolute GHG emissions by 2030 (striving for 30%) and a 70% reduction by 2040 (striving for 80%).
Along with these revised targets, the IMO introduced a series of measures including lifecycle GHG emissions accounting for marine fuels. Each bunker fuel will come with a Fuel Lifecycle Label (FLL) with information regarding the fuel type, the feedstock and processes used in its production, its GHG emissions factor and other sustainability related data. Biofuels are included in these guidelines; specifically, for biofuels that are certified by an international certification scheme to have a lifecycle GHG footprint up to 33 g CO2e/MJ (less than 65% of MGO), the IMO will allow the use of the certified GHG footprint for well-to-wake emissions calculations of the vessel.
The 33 gCO2e/MJ limit was certainly not randomly chosen; it is above the lifecycle carbon footprint of biodiesel (Fatty Acid Methyl Esters – FAME) and renewable diesel (Hydrotreated Vegetable Oil – HVO) as calculated for most feedstock options – such as soybean and yellow grease respectively [1–5] – which makes them eligible for use. Biomethanol is also eligible when produced from biomass or agricultural waste [6, 7].

Figure 1: Well-to-Wake carbon emissions of conventional and alternative marine fuels (gCO2eq/MJ). Source: ABS [8]
This interim guideline paves the way for the adoption of biodiesel as bunker fuel, which is expected to happen in progressively increasing blends with HFO, VLSFO or MGO. During the last few years, multiple trials have shown that biodiesel is a good drop-in fuel. Anecdotal evidence has shown that biodiesel-fuel oil blends have little to no impact on combustion or machinery wear and maintenance needs. The oxygen content of biodiesel (FAME) can slightly increase the NOx emissions of the main engine, but data from the same trials have shown that the increased NOx is not enough to affect the Tier II/III certifications of the engines (g NOx/kWh). The only documented drawback of using biodiesel is its storage: due to its oxygen content, biodiesel tends to promote bacterial growth that form a sludge when stored over long time periods (months). Therefore, implementing a fuel condition monitoring and treatment protocol is recommended.
These issues can be easily solved with the use of renewable diesel (HVO). HVO is a superior fuel to biodiesel primarily because of its lack of oxygen content and aromatic compounds, which result in excellent storage stability and reduced particulate matter formation from combustion. It is also free of sulfur and has a higher cetane number than both petroleum diesel and biodiesel [9].
However, both biodiesel and HVO are currently limited in production capacity worldwide. Recent data from the International Energy Agency (IEA) show that in 2019 the global biodiesel production was 41 billion L, while the global HVO production was just 7 billion L [10–14]. These production numbers are projected to grow to 46 billion L of biodiesel and 17 billion L of HVO by 2025 [10–14], which indicates an acceleration of HVO production due to global demand. The annual fuel consumption in global shipping is estimated at 330 million metric tons [15], therefore the combined global biodiesel and HVO production in 2025 would only be able to cover about 17% of the global shipping fuel demand (56 million metric tons).

Figure 2: Global biodiesel consumption (2010–2020) in million liters/year. Source: IEA [16]
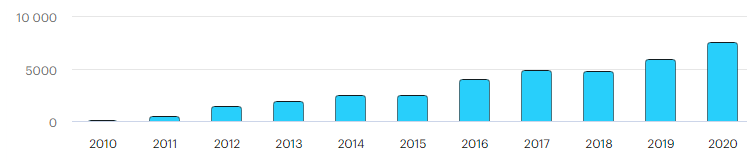
Figure 3: Global renewable diesel consumption (2010–2020) in million liters/year. Source: IEA [17]
Upscaling the production of biodiesel and HVO is expected to reduce their cost, which is currently high, especially for HVO. Recent data from the Port of Rotterdam show that a B30 blend (30% biodiesel – 70% VLSFO vol.) is sold at a 23% premium compared to VLSFO, and a B20 blend is sold at a 17% premium [18]. HVO – fuel oil blends are expected to be somewhat more expensive, but it is difficult to make accurate estimates due to the lack of data or experience from using HVO as bunker fuel. The expected demand for biodiesel and HVO from global shipping creates business opportunities in the production, distribution, and bunkering space.
The recent uptake of methanol as an alternative fuel for container carriers and tankers is expected to create a demand for biomethanol in the future. Biomethanol is the exact same compound as methanol but produced from renewable feedstock, which makes it a true drop-in fuel and fully compatible with the latest dual fuel methanol engines. Global methanol production is high, as 111 million metric tons of methanol were produced in 2022, but less than 1% of this was made from renewable sources [19]. However, over 60% of global methanol production is currently used in the chemical industry. Therefore, the demand for methanol and biomethanol as bunker fuel will necessitate an increase in global production, which will create additional business opportunities. Bunker methanol is currently offered at prices similar to HFO, but biomethanol is expected to be somewhat more expensive.

Figure 4: Cost comparison of renewable methanol with conventional fuels (USD/GJ). Source: IRENA [20]
LNG-fueled vessels will have the option of using renewable LNG or biomethane in the future. Renewable LNG is a pipeline-quality gas that is fully interchangeable with natural gas and thus can be used as a drop-in fuel in gas combustion processes. Renewable LNG can be produced from various sources, including livestock waste, landfills, wastewater sludge, food waste and other organic waste operations [21]. Biomethane is a further processed product, which has been cleaned and conditioned to remove non-methane species. LNG-fueled vessels can directly use renewable LNG or biomethane as drop-in fuels. However, LNG carriers that consume boil-off gas for propulsion will likely need to be fitted with additional fuel storage tanks onboard to utilize renewable LNG or biomethane.
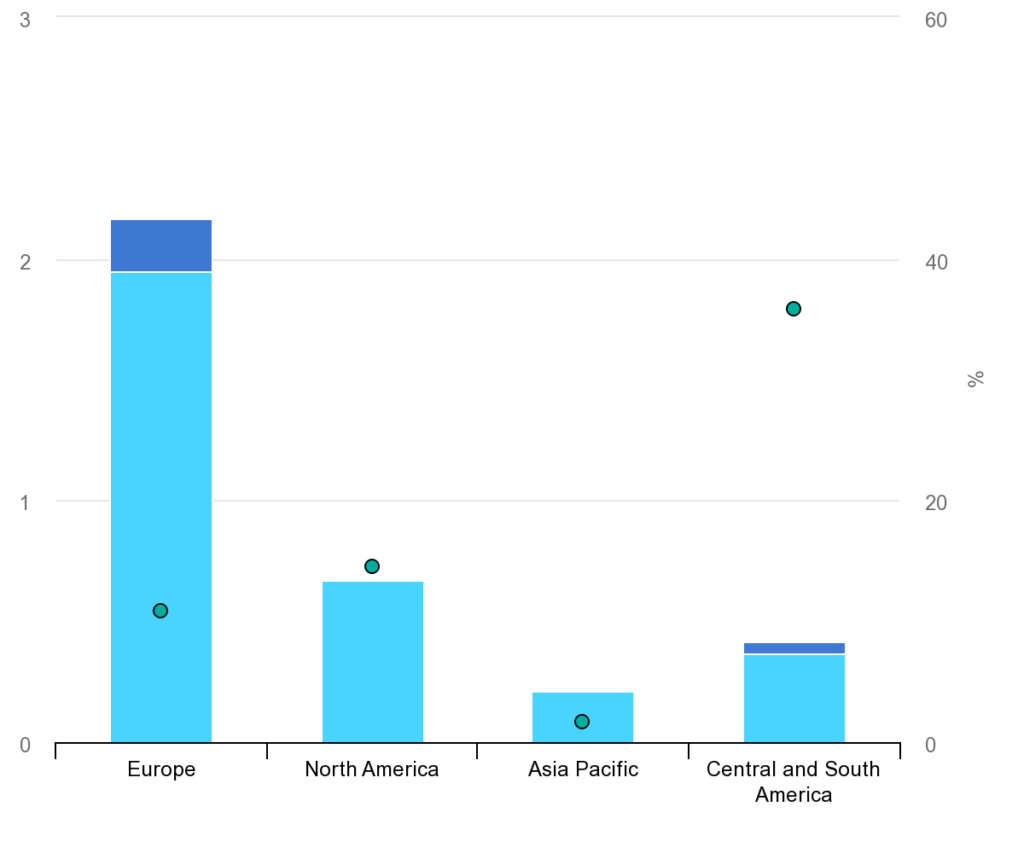

Figure 5: Biomethane production (in Mtoe) and share of total biogas production that is upgraded in selected regions, 2018. Source: IEA [22]
The latest developments from MEPC 80 have shown a convergence between IMO and the Fuel EU Maritime regulation. The Fuel EU has mandated reductions in well-to-wake GHG emissions accounted on a lifecycle basis that are made progressively stricter from 2025 to 2050. In this progression, the 33 gCO2e/MJ limit for biofuels stated by the IMO aligns with the 2045 target of the EU. This means that a biofuel that has a lifecycle GHG intensity of less than 33 gCO2e/MJ can start to be used in progressively higher blends with fossil fuels so that it becomes the sole fuel of the vessel by 2045. This strategy will likely satisfy all the requirements of the IMO and EU, both in terms of reducing the vessel’s carbon intensity (CII) and her absolute GHG emissions.

Figure 6: FuelEU Maritime annual average carbon intensity reduction compared to 2020. Source: European Council [23]
The only discrepancy between the IMO and the Fuel EU framework seems to be the accounting of potentially negative carbon footprint of e-fuels (or green fuels). The IMO sets a minimum lifecycle carbon footprint at zero (carbon neutral), whereas the Fuel EU allows for negative carbon footprint values. Lastly, the Fuel EU mandates the use of 2% e-fuels by 2034.
The recent developments of MEPC 80 set the stage for meaningful reductions in the carbon footprint of the global fleet. In fact, describing the 2030 and 2040 IMO targets in terms of absolute GHG emissions reduction will require effective measures in the forms of alternative fuels, energy efficiency technologies, and operational measures. Also, the guidelines on lifecycle GHG emissions accounting for marine fuels mark a paradigm shift in the way vessel GHG emissions are viewed. Instead of focusing on tank-to-wake GHG emissions we will have to focus on well-to-wake values (lifecycle). Also, instead of thinking just in terms of CO2, we will have to account for the global warming potential of CH4 and N2O as well. Biofuels are an important lever in the effort of global shipping to reduce its carbon footprint because they will enable the continued use of existing power generation and propulsion systems with minimal to no changes.
References:
- Xu H., et al., Environ. Sci. Technol. 2022, 56, 12, 7512–7521
- Chen R., et al., Bioresource Technology 2018, 251, 249-258
- ERIA Study team (2022), ‘Well-to-Tank CO2 Emission from Biofuels’, in Morimoto, S., et al., ERIA Research Project Report FY2022 No. 16, Jakarta: ERIA, pp.4-23.
- Seber G., et al., Biomass and Bioenergy 2014, 67, 108-118
- Tan E. C. D., et al., Environ. Sci. Technol. 2021, 55, 7561−7570
- De Jong, M., et al., “Methanol Carbon Footprint and Certification”, 2022, IMPCA
- Corbett, J. J., et al., “Life Cycle Analysis of the Use of Methanol for Marine Transportation”, U.S. MARAD, 2018
- ABS MEPC 80 Brief
- McCaffery, C., et al., “Effects of hydrogenated vegetable oil (HVO) and HVO/biodiesel blends on the physicochemical and toxicological properties of emissions from an off-road heavy-duty diesel engine”, Fuel, 323, 2022, 124283
- IEA (2020a), Monthly Oil Data Service (MODS), August 2020
- IEA (2020b), Oil Information (database)
- IHS Markit (2020)
- Biofuels market; MAPA (2020)
- Agroenergia; US EIA (2020)
- U.S. Department of Transportation, Maritime Administration, 2020
- IEA: Renewables 2021 Analysis and forecast to 2026
- IEA: Renewables 2021 Analysis and forecast to 2026
- Ship & Bunker News Team, March 21, 2023, shipandbunker.com
- European Technology and Innovation Platform, Bioenergy, 2022
- IRENA: Innovation Renewable Methanol
- IEA: Outlook for biogas and biomethane
- EU Fit for 55